@ Brown University

What Interests Us: How Do Organisms Respond to Stress?
At its simplest level, the living cell can be thought of as a compartmentalized microreactor that constantly senses its environment, including stress signals, and through a series of well-coordinated unit operations, generates an output that leads to adaptation, and ensures survival even under adverse conditions. Given the extraordinary complexity of the environment, the stress response is, not surprisingly, equally complicated. The overarching goal of the Deaconescu Laboratory is to unravel basic mechanisms of stress response using an integrative approach, combining protein engineering, structural biology and biochemistry in bulk with single-molecule biophysics, molecular genetics and functional studies in cells and animal models. Our current focus lies on cellular responses elicited by DNA damage.
Interplay of DNA Repair and Transcription: From Structures to Mechanisms
The double-helical nature of DNA, the length of genes, and the ubiquitous presence of endogenous and exogenous DNA-damaging agents pose significant topological and information processing challenges to the cell. DNA serves as a track for a variety of essential cellular machines, including the DNA replication and transcription machineries that scan or read the chromosome. Our overall goal is to understand how DNA repair pathways interface with other cellular processes. To this end, we are focusing on a specialized subpathway of nucleotide excision repair (NER) called transcription-coupled DNA repair (TCR). TCR is triggered by the stalling of RNA polymerase molecules at certain DNA lesions, such as UV-induced damage. In TCR, as in NER, repair is achieved through a "cut and patch" mechanism, in which excision of a short oligonucleotide containing the DNA damage is followed by resynthesis and gap filling. Unlike in genome-wide NER, the initial recognition of the DNA damage is mediated by RNA polymerase itself (rather than dedicated repair machinery), which stalls and serves as a beacon for recruitment of transcription-repair coupling factors. These, in turn, remodel or dissociate RNA polymerase transcription complexes and preferentially recruit NER proteins to the damaged site.
TCR exists in both prokaryotes and eukaryotes, and, in humans, has been associated with a variety of syndromes, such as UV-sensitive syndrome, DeSantis Cacchione, and the better-known progeroid (accelerated-aging) Cockayne syndrome, characterized by severe, multi-systemic developmental and neurological defects. In ongoing experiments, we are dissecting the complicated mechanochemistry of transcription-repair coupling factors with the overall goal of understanding how these proteins, part of a large family of dsDNA translocases, utilize the energy of ATP hydrolysis to exert mechanical work to remodel their substrates and recruit NER proteins.
Work in this area has been highlighted by Molloy, S. Nature Rev. Microbiology (2006) 4, 240-241, Faculty of 1000, the Scientific Bulletin of the Advanced Photon Source (Argonne National Laboratory), a Rockefeller and Cornell University press release.



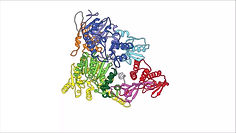
The General Stress Response and Regulation of RpoS Turnover by the ClpXP Machine


The dissociable promoter recognition subunit RpoS is the master transcriptional regulator of the general stress response in gamma-proteobacteria, and plays key roles in the virulence of many pathogens, including human, plant and animal pathogens. Under certain conditions, such as at the transition from the logarithmic to the stationary phase or in the presence of stress signals, RpoS redirects the core RNA polymerase machinery to a subset of promoters to reprogram transcription. However, intracellular RpoS levels are not steady: they are low in actively dividing cells, and substantially increased upon entering the stationary phase or upon encountering stress. To achieve proper regulation, there is tight control over RpoS levels, with the major point of regulation occurring at the level of RpoS proteolysis by the ATP-dependent ClpXP machine. Our central focus is to understand the mechanisms of RpoS proteolysis by ClpXP as well as its regulation by an emerging group of proteins collectively called anti-adaptors. In order to be degraded, RpoS is presented to ClpXP by a unique, highly specific adaptor called RssB, which acts catalytically, without being degraded. In turn, RssB itself is regulated by interactions with stress-specific anti-adaptors. Our work focuses on the structure and function of three-anti-adaptors: IraD (induced by oxidative stress and DNA damage), IraM (induced by magnesium starvation) and IraP (induced by phosphate starvation). These anti-adaptors share no sequence homology, and only weak homology with protein of known structure, warranting a structural biology effort aimed at deciphering the underlying mechanisms of RssB recognition. Overall, this work not only brings fundamental, mechanistic insights, but will also open the way to the development of novel antibacterials that could target ClpXP directly, or, adaptor/anti- adaptor interfaces. The RpoS regulon has been reported to comprise up to 10% of the Escherichia coli genome, and RpoS itself plays important roles in bacterial persistence, host-pathogen interactions and biofilm formation, which underlies 80% of all infections.
Work in this area has been highlighted in a Brown University press release (2019).
Cytoskeletal Structures and Regulation
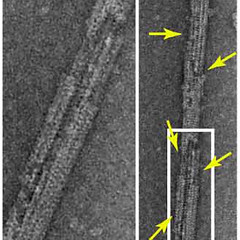
Cytoskeletal regulation represents a third focal point of research in our lab. We have collaborated in this area with the labs for Doro Kern (Brandeis University), Antonina Roll-Mecak (NIH) and Bruce Goode (Brandeis University). Our interests has mainly lied in the regulation of microtubule dynamics by post-translational modifications and severing enzymes, such as spastin. We have applied the methods of small-angle X-ray scattering and transmission electron microscopy to tackle questions in this area.
Work in this area has been previewed by Kull & Sloboda, Cell (2014) 157(6): 1255-12256, highlighted in ACS Chemical Biology (Dahlmann HA, 2014), Science (Hurtley S, 2018) and Developmental Cell (Akhmanova A, 2018; Stavoe AKH & Holzbaur ELF, 2018), an NIH press release, recommended by Faculty of 1000 and featured on several news outlets, including EurekAlert!, and Nanowerk.
Our Collaborators:
-
Dorothee Kern Group (HHMI and Brandeis University)
-
Antonina Roll-Mecak Group (NIH and NINDS)
-
Michelle Wang Group (HHMI and Cornell University)
-
Susan Gottesman Group (National Cancer Institute)
-
Sue Wickner Group (National Cancer Institute)
-
Dmitry Lyumkis Group (The Salk Institute)
-
Brenda Rubenstein Group (Brown University)
Funding

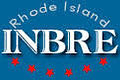


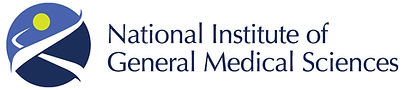